PALEOCLIMATE RECONSTRUCTIONS AND DATA
Many of PARC’s research projects have involved the use of our 1,000-year database of tree-ring data and reconstructions of the climate and hydrology of the past millennium. Below we describe the features, applications and limitations of these data, and discuss the incorporation of paleoclimate data into projects. Be sure to click on the video courtesy of the Prairie Climate Centre!
APPLYING OUR KNOWLEDGE OF PAST CLIMATE AND WATER LEVELS
At PARC, information on the climate of the Prairie Provinces over the past millennium complements the data we obtain from weather stations and climate models. This ‘paleo’ perspective is an effective starting point for informing stakeholders about the variability of our climate. Many people relate more easily to the climate that actually has occurred as opposed to the virtual climate simulated by models. Managers of water, land, and infrastructure have asked us to distinguish between anthropogenic climate change and the natural variability of our regional hydroclimate. The public are more likely to support adaptation plans and actions when a signal of anthropogenic climate change has clearly emerged from a background natural variability. A significant question that occurs to both scientists and practitioners is the extent to which global warming has caused the regional climate to depart from the historical climate regime to which social and ecological systems are adapted.
Charron (2014: 67) advised users of climate data:
Understanding that natural variability exists in the recent climate is
important for a number of reasons. First, it can serve to remind users
that for future climate projections, they are not given one future but a range
of possible future climates even for a single emission scenario. Moreover,
information on the range of past climate can be a good starting point to
evaluate the need for adaptation and, in some cases may be informative
enough to make decisions, without the need to rely on future climate
projections.
The fact that model projections give “a range of possible future climates even for a single emission scenario” is due in large part to the irreducible internal variability of the climate system. Various authors (e.g., Deser et al. 2014; de Elia and Cote, 2010; Music et al., 2012; Knutti and Sedláček 2012) have emphasized that, despite large increases in computing capacity and advances in the numerical modeling of global and regional climate, there has not been a commensurate improvement in the precision of the models. Deser et al. (2012) concluded:
Natural climate variability poses inherent limits to climate predictability…
contributes substantial uncertainty to temperature and precipitation trends
over North America, especially in winter at mid and high latitudes… [It] is
unlikely to be reduced as models improve…
The mid latitudes of North America include Canada’s western interior and winter is the season that produces most of our water supply. Barrow and Sauchyn (2019) were able to confirm that natural climatic variability is the dominant source of uncertainty in the projection of the future climate of western Canada. The following diagram from their paper illustrates that internal variability accounts for about 90% of the total variance among climate model projections of future precipitation over the next few decades. The variance explained by differences among models is relatively small and consistent over time. Assumptions about anthropogenic emissions of greenhouse gases (i.e., the GHG scenario) become a factor only after mid-century and account for only about 10% of the total variance at the end of the century; natural variability remains dominant.
The fraction of total variance in future projections of winter precipitation explained by internal variability (orange), model uncertainty (blue) and GHG scenario uncertainty (green) for western Canada (from Barrow and Sauchyn, 2019)
This finding, concerning the inherent limits to climate predictability in western Canada, suggests that climate change risk assessment requires knowledge of both the regional consequences of global warming and the natural variability of the regional climate regime. Instrumental weather records have tracked the variability of the climate of western Canada for the past 140 years, but because this coincides with the period of greenhouse gas forcing of the global climate, weather data represent both natural climate variability and anthropogenic climate change, or as Hughes and Diaz (2008) put it:
The instrumental weather record is neither an adequate nor unbiased sample
of the nature, range and character of natural climate variability; the climate
system has been reconfigured.
The biases they refer to are the trends imposed by global warming, and the inadequacy relates to a record length that is too short to capture the full range and character of climate variability. The longest weather records in our region provide a good sampling of inter-annual variability, which has been linked to influence of the El Niño South Oscillation (ENSO) in the tropical Pacific Ocean. Decadal scale cycles also characterize our climate. Instrumental weather records provide a relatively small sample of these cycles lasting decades.
This decadal mode of climate variability is very important for detecting anthropogenic climate change and for managing natural resources. Whereas annual or seasonal mean values factor in to the design of water management structures, inter-annual to decadal variability and extreme hydrologic events, rather than long-term trends in water yield, present most of the challenge for managing watersheds and operating water conveyance and storage structures. Decades long trends in precipitation and water levels, representing part of a decadal cycle, can be mistaken for climate change. Also, the internal variability and external forcing of climate interact such that the anthropogenic global warming can be amplified or offset by natural factors, for example with release of heat from the tropical oceans during an El Nino event. Climate modeling can be challenged to capture this natural (internal) variability, especially at regional scales, with implications for the use of climate projections. As an example, a study of the Colorado River (Harding et al., 2012) concluded that unforced (internal) variability is the largest source of uncertainty for the projection of future flows. A random or chaotic component dominates the projected change in streamflow, and climate models differ substantially in terms of the phasing of low-frequency (decadal) variability.
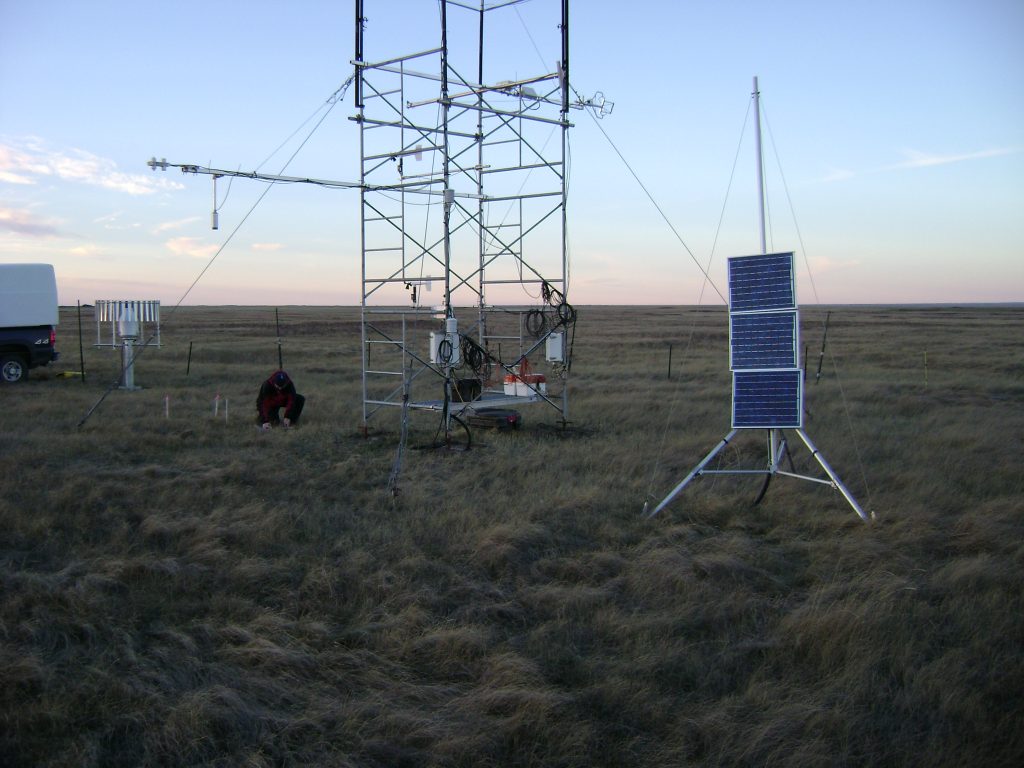
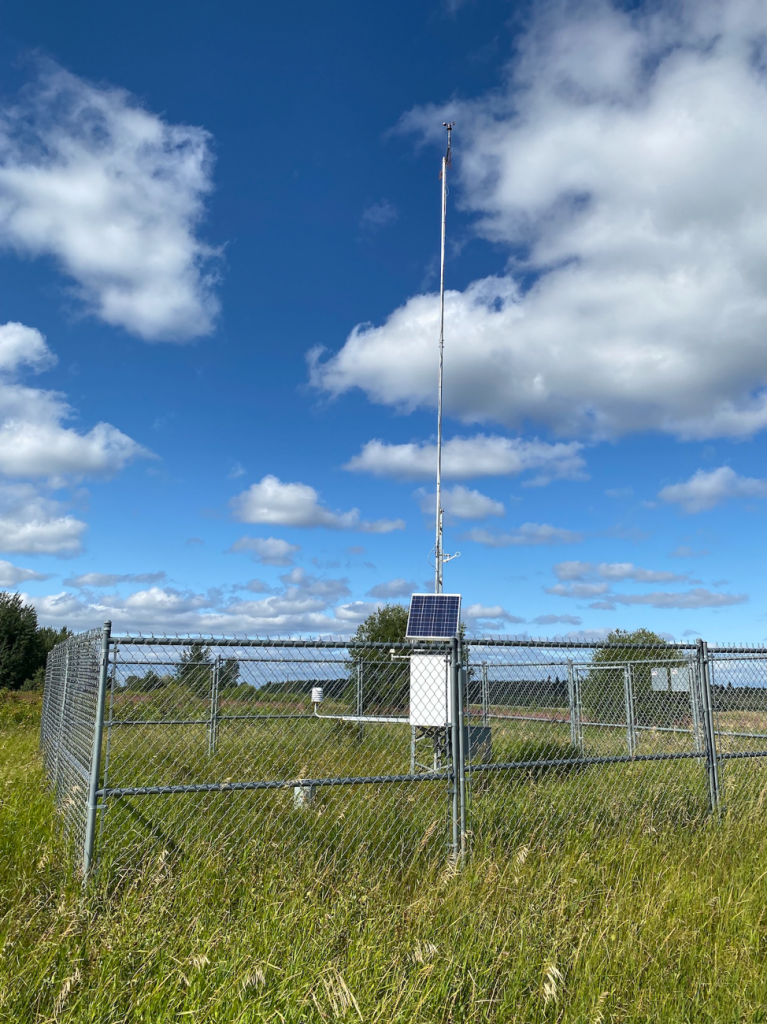
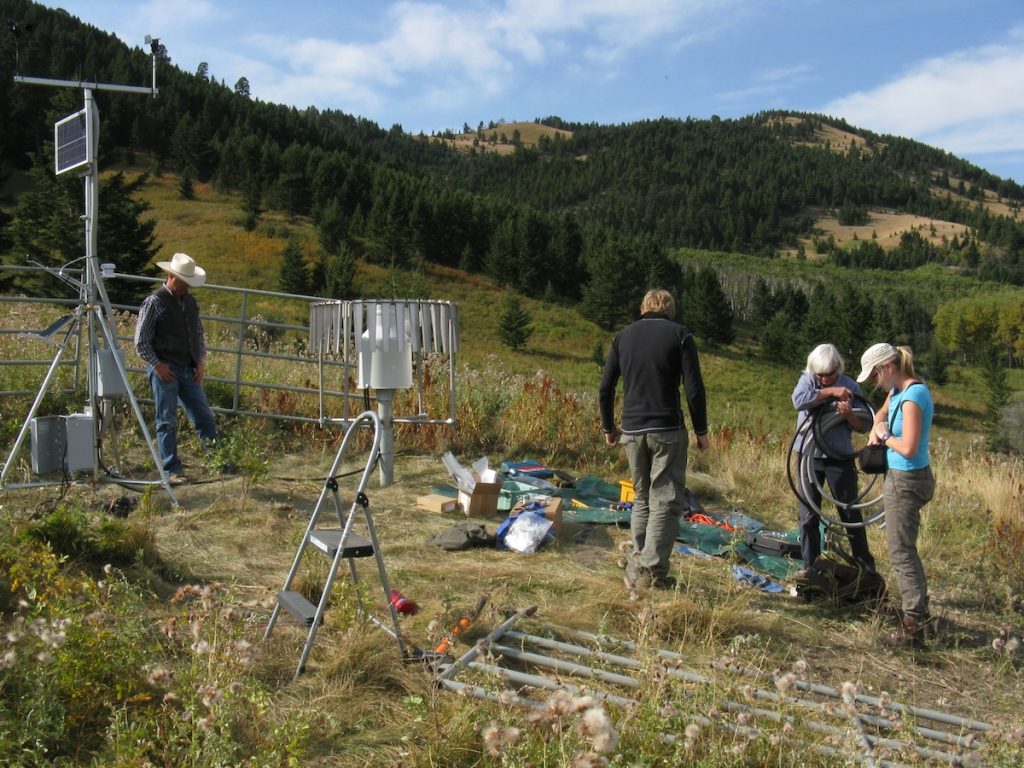
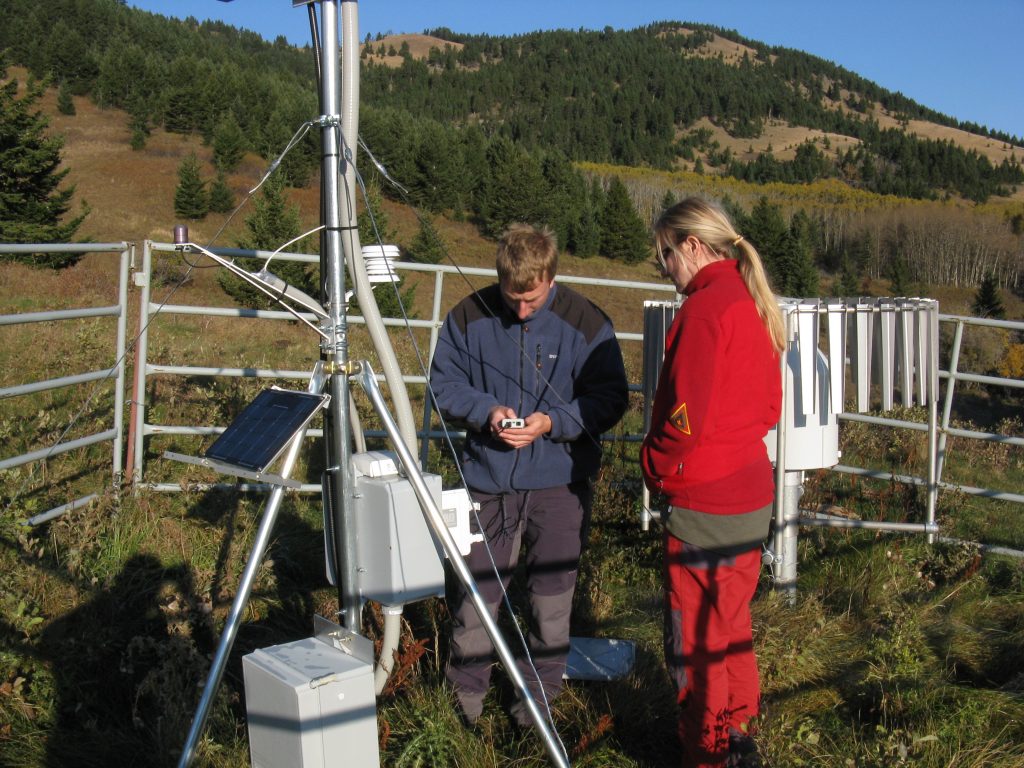
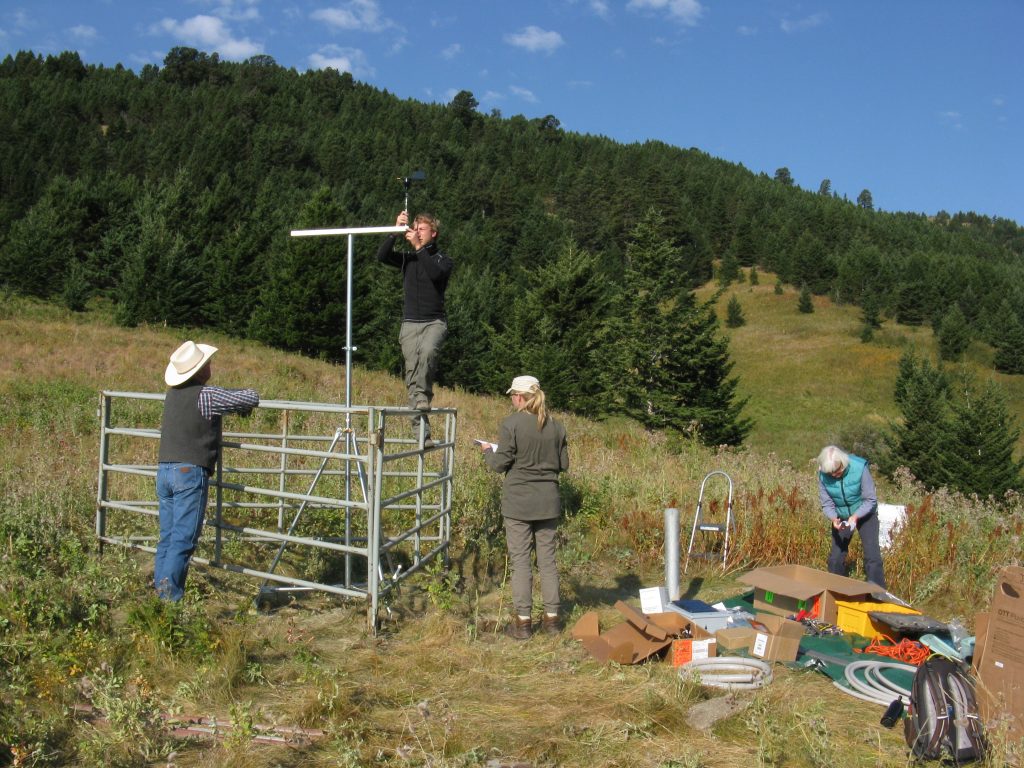
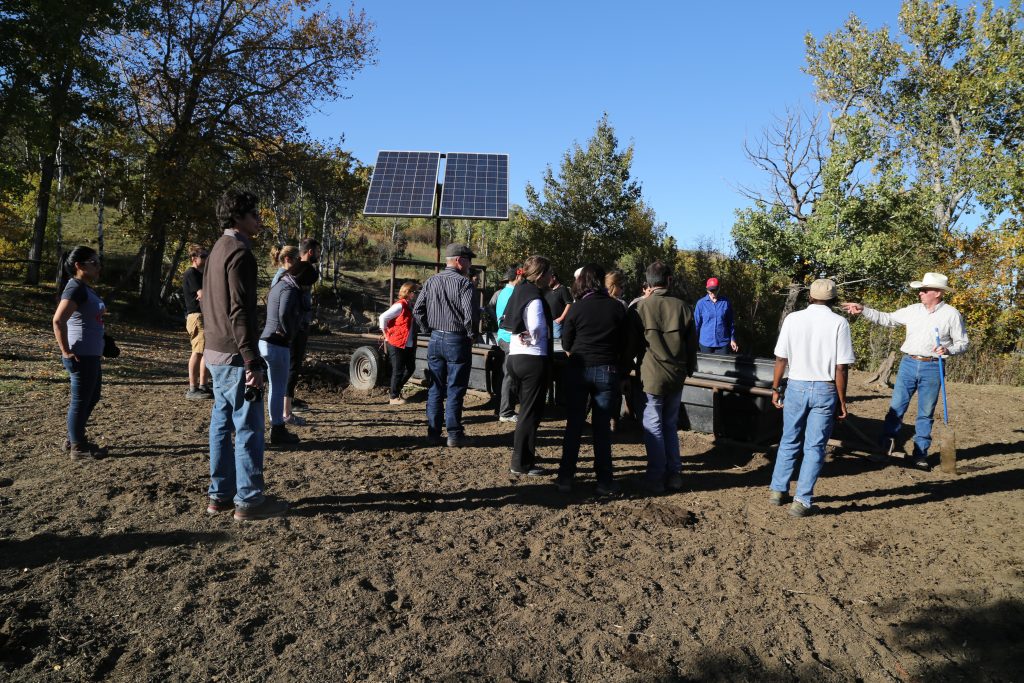
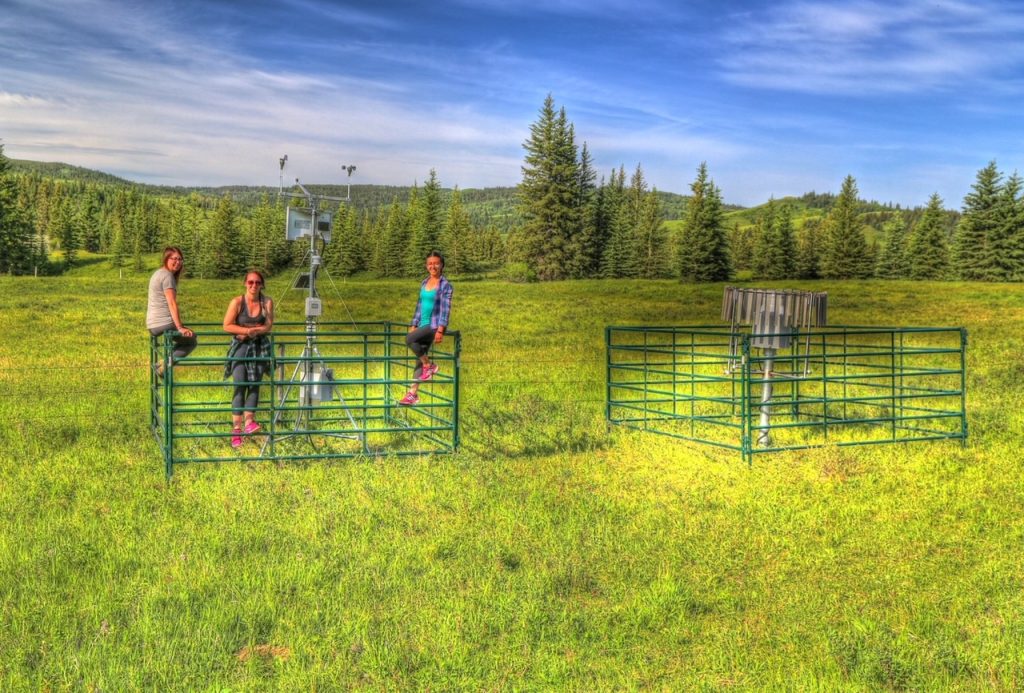
PRAIRIE PALEO-HYDROCLIMATE FROM TREE-RINGS
Research on the Earth’s past climate has made a pivotal contribution towards our understanding of climate change. Climate reconstructions are the empirical evidence for the amplitude and forcing of the Earth’s climate over geological time, and the unusual nature of recent climate change. Knowledge of past climate places constraints on the range of climate conditions projected by models. Thus, paleoclimate records provide a historical context for the interpretation of instrumental weather data and projections of future climate. Whereas model projections may fall outside the climate of instrumental ‘data rich’ decades, they may not be anomalous relative to the climate of past millennia.
The climate and hydrology of the past is preserved in biological and geological archives. Ecosystems and sediments act as recorders of environmental change enabling the reconstruction of past climate variability on seasonal, annual and century-long time scales. On the northern Great Plains, changes in climate are evident from the shifting of regional ecosystems, fluctuations in lake level and salinity, patterns of tree rings, and the mobility of sand dunes. In this dry environment, these climate proxies tend to be most sensitive to fluctuations in the soil and surface water balance.
Whereas the contents and properties of lake sediments provide a long and continuous record of environmental change, tree rings are the preferred proxy of the climate of the last millennium, because they are a source of both hydroclimatic data and a chronology with absolute annual resolution. The increment of annual tree growth is determined by the availability of moisture at dry sites and by heat in stands of trees at high elevations or high latitudes. The mathematical relationships between standardized tree-ring widths and data from water gauges and weather stations are applied to the tree-ring data to reconstruct the annual and seasonal hydroclimate each year over the entire length of the tree-ring record. Tree rings from living and dead trees that were growing at the same time for at least a few decades can be cross-dated, such that calendar years can be transferred from the living to the dead trees extending tree-ring chronologies over centuries and millennia.

The University of Regina Tree-Ring Lab, based at PARC, has collected increment cores from old living trees and disks from dead trees at almost 200 sites in the boreal, montane, and island forests of the Prairie Provinces and adjacent areas. This network of tree-ring chronologies has been the basis for reconstructing annual and seasonal water levels, precipitation, moisture indices, and temperature at various locations over the past 300-1000 years.

For the reasons described on this page, many of PARC’s research projects have involved the use of our database of tree-ring data and reconstructions of the climate and hydrology of the past millennium. Following are links to pages that provide brief descriptions of some of these projects and how the use of paleo-hydroclimatic data contributed to assessments of regional climate change, impacts and risks, and the evaluation of adaptation strategies.
PARC has developed a database of Canadian Prairie Provinces’ climate over past centuries. This product makes PARC unique among Canadian organizations providing climate services. It has proven to be a very useful complement to data derived from weather stations and climate models, and popular among stakeholders in our region since proxies of past climate capture natural and real – as opposed to modeled – variability. Because the monitoring of weather since the late-19th century has coincided with the period of greenhouse gas forcing of the global climate, weather data capture both natural climate variability and anthropogenic climate change. On the other hand, proxy climate data that predate the industrial revolution record only natural climate variability (e.g., Figure 1). Recent research has demonstrated that the natural variability of prairie climate is a major source of uncertainty in the projection of regional climate change. Also, a significant question that occurs to both scientists and practitioners is the extent to which global warming has caused the regional climate to depart from the historical climate regime to which social and ecological systems are adapted.
Our Paleo Projects / Data in Action
Please click on the boxes below for visualizations of our paleoclimate data.
The Paleoclimate of SW Alberta
Past Climate in the Churchill River Basin
Requesting Paleoclimate Data from PARC
If you or your organization has a Prairies project that may benefit from, or require the use of, hydro-climate data, please contact us to discuss how we may be of assistance. We may have a data set available or be able to assist in collecting data to support your needs. Please note that our data are not accessible to non-technical users who are not familiar with processing large data sets.
References
Barrow, E., and D. Sauchyn (2019). Uncertainty in climate projections and time of emergence of climate signals in the western Canadian Prairies. International Journal of Climatology, 39(11): 4358-4371.
Charron, I. (2014) A guidebook on climate scenarios: using climate information to guide adaptation research and decisions. Ouranos (online): www.ouranos.ca
Deser, C., R. Knutti, S. Solomon, and A.S. Phillips. Communication of the role of natural variability in future North American climate. Nature, Climate Change 2: 775-779.
Deser, C., A.S. Phillips, M.A. Alexander, and B.V. Smoliak (2014). Predicting North American climate over the next 50 years: uncertainty due to internal variability. Journal of Climatology, 27: 2271-2296.
de Elia, R., and H. Cote (2010) Climate and climate change sensitivity to model configuration in the Canadian RCM over North America. Meteorologische Zeitschrift, 19(4): 1-15.
Hughes, M.K., and H. Diaz (2008) Climate variability and change in the drylands of western North America. Global Planetary Change, 64: 111-118.
Harding, B.L., A.W. Wood, and J.R. Prairie (2012) The implications of climate change scenario selection for future streamflow projection in the Upper Colorado River Basin. Hydrology and Earth System Sciences, 16(11): 3989-4007.
Knutti, R., and J. Sedláček (2012) Robustness and uncertainties in the new CMIP5 climate model projections. Nature, Climate Change: 3(4): 1-5.
Music, B., D. Caya, A. Frigon, A. Musy, R. Roy, and D. Rodenhuis (2012) Canadian Regional Climate Modeal as a Tool for Assessing Hydrological Impacts of Climate Change at the Watershed Scale. In: Berger, A., F. Mesinger, and D. Sijacki (eds.) Climate Change. Springer, Vienna: https://doi.org/10.1007/978-3-7091-0973-1_12.